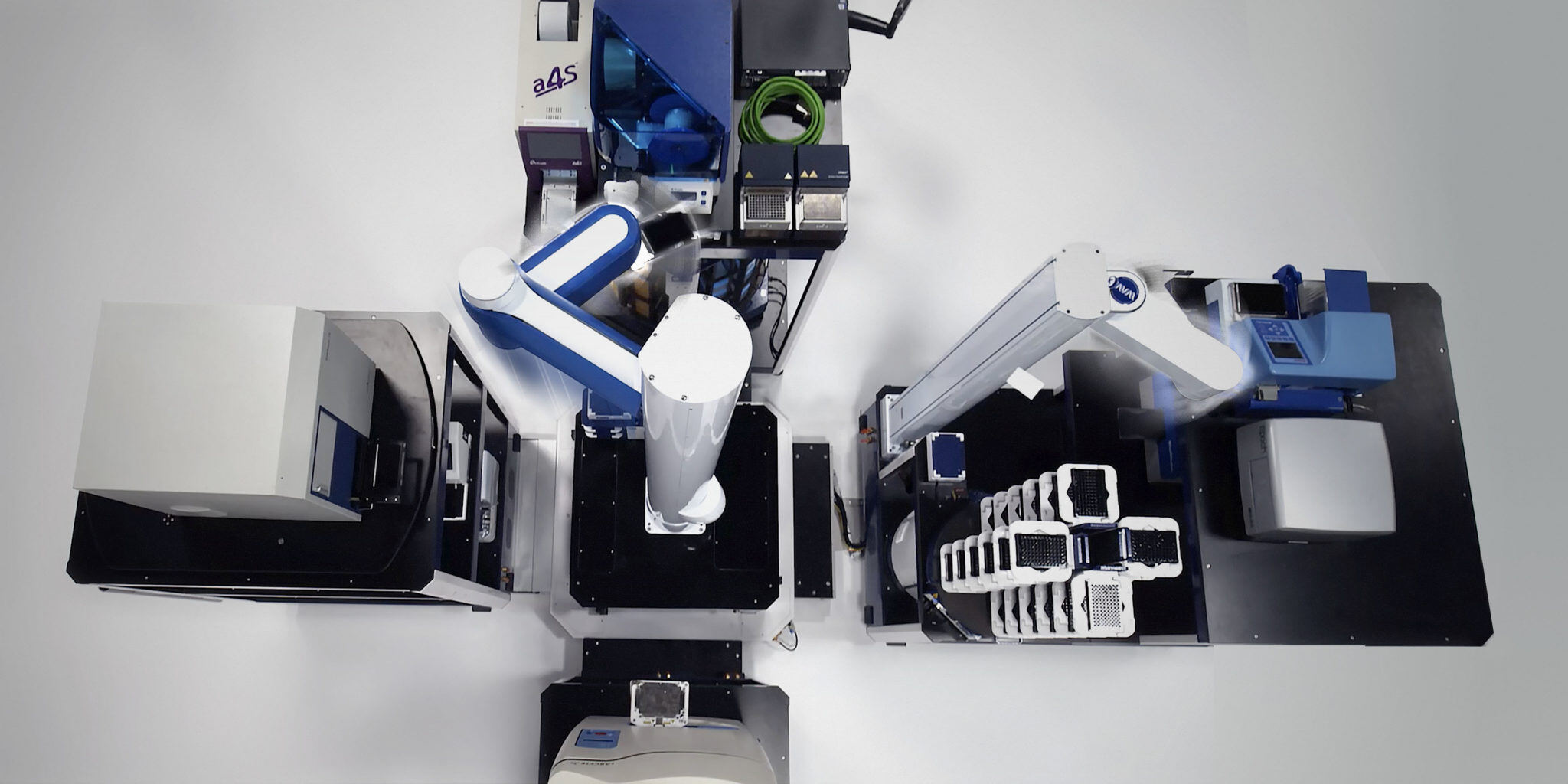
Introduction
Laboratory automation has revolutionized modern laboratories, enhancing efficiency and accuracy in various lab operations. Here, we provide an overview of the different types of laboratory automation and their significance in today's lab settings.
Understanding the different types of laboratory automation is crucial as it allows labs of different scales and settings to choose the most suitable automation type. This knowledge can lead to improved productivity, accuracy, and safety in laboratory operations. Automation can reduce manual errors, streamline workflows, and increase the reproducibility of results, making it an essential component in both research and clinical laboratories.
The Goal of Laboratory Automation
The primary goal of laboratory automation is to free up scientists' time, reduce the chance of human error, and increase the throughput of experiments. Automation encompasses a wide range of technologies, from simple devices that automate individual tasks to complex robotic systems that control entire workflows. It can be applied to various laboratory functions, including sample preparation, analysis, data management, and reporting.
Types of Laboratory Automation
Manual Systems
Manual workflows that employ limited numbers of automated devices (i.e., electronic pipettors, basic spectrophotometers) are still prevalent in many laboratories today. These “manual systems” rely upon significant human intervention, but they can be useful for low-throughput labs or for tasks that are difficult to automate. Manual systems involve basic instruments that enhance precision and repeatability but rely heavily on human operation. While manual systems do not represent true lab automation, these tools remain essential for many labs, especially those with limited resources or specific research needs.
Stand-Alone Automation (Modular)
Stand-alone or modular laboratory automation systems are designed to automate a single process or a group of related processes. These systems offer flexibility as they can be used independently or combined with other systems. An example of this is an automated pipetting system, which can significantly increase accuracy and efficiency in liquid handling tasks. Modular systems are advantageous because they can be scaled up or down based on the lab's needs. For instance, a lab might start with an automated plate reader and later integrate it with robotic arms for sample handling, creating a more comprehensive automated workflow.
Total Laboratory Automation (TLA)
Total Laboratory Automation (TLA) systems represent the highest degree of automation. These systems integrate several different processes and instruments into a single, coordinated system. High-throughput labs, such as drug discovery and clinical diagnostic labs, often use TLA to handle large volumes of samples efficiently. TLA systems can automate the entire laboratory process, from sample receipt to result reporting. They are particularly beneficial in environments where speed and accuracy are critical, such as in large research facilities and hospitals. TLA systems reduce turnaround times and minimize the risk of contamination or human error.
Technologies Used in Laboratory Automation
Robotics
Robotics play a crucial role in lab automation. Robotic systems can carry out repetitive tasks, such as moving samples between instruments, with high precision and speed. This increases efficiency and reduces the risk of repetitive strain injuries for lab personnel. Robotic systems can be programmed to perform complex sequences of operations, ensuring consistency and reliability in experimental procedures. For example, robotic arms can automate the preparation of hundreds of samples, which is particularly useful in high-throughput screening and drug discovery.
Artificial Intelligence
Artificial Intelligence (AI) enhances lab automation by enabling more intelligent and adaptable systems. For instance, AI algorithms can optimize scheduling of tasks on an automated platform to maximize throughput. AI can also analyze data in real-time, providing insights that can improve experimental outcomes. In diagnostics, AI can assist in interpreting complex data, such as imaging or genetic information, leading to faster and more accurate diagnoses. AI-driven automation can adapt to changing conditions and learn from previous experiments, continuously improving its performance.
Automated Analyzers
Automated analyzers are instruments that can carry out a series of tests unattended. These devices are commonly used in clinical labs for tasks such as blood testing. Automated analyzers can process large numbers of samples quickly and accurately, reducing the workload on lab staff. They can perform various assays, including biochemical, immunological, and molecular tests, with minimal human intervention. Automated analyzers ensure standardization and reproducibility, which are critical for clinical and regulatory compliance.
3 Stages of Laboratory Automation
1.- Initial Assessment and Planning
The first stage in implementing lab automation involves assessing the lab's needs and setting goals. This step is crucial for understanding what processes to automate and what type of automation system would be most beneficial. During this phase, labs must evaluate their current workflows, identify bottlenecks, and determine the potential return on investment for automation. A thorough needs assessment helps in selecting the right technology and justifying the investment to stakeholders.
2.- Implementation and Integration
Once a plan is in place, the next step is to implement and integrate the automation system into the lab workflow. This stage involves both technical setup of the equipment and training of staff. Integration can be complex, requiring coordination between different instruments and software systems. Effective implementation also involves validating the automated processes to ensure they meet regulatory standards and lab-specific requirements. Training staff to use and maintain the new systems is critical for a smooth transition and long-term success.
3.- Monitoring and Optimization
After implementation, it's important to continuously monitor the system and adjust as needed. This ongoing process ensures that the automation system remains efficient and effective over time. Regular maintenance and updates are necessary to keep the system running smoothly. Labs should also collect performance data to identify areas for improvement and to justify further investments in automation. Continuous optimization helps in adapting to new challenges and leveraging advancements in technology.
Conclusion
Understanding the different types of laboratory automation systems is key to choosing the right system for any given lab. By selecting the appropriate level of automation, labs can improve efficiency, increase throughput, and allow scientists to focus on more complex tasks. Whether it's a manual system, a modular setup, or a fully integrated TLA system, each type of automation offers unique benefits that can enhance a lab's operations. The right automation strategy can transform lab productivity, reduce errors, and ultimately lead to better scientific outcomes.
Laboratory automation is a powerful tool that can significantly impact the efficiency, accuracy, and safety of laboratory operations. By understanding and implementing the appropriate types of automation, labs can stay ahead in a competitive and rapidly evolving scientific landscape.
Revision: BL-DIG-240701-01 RevB - Laboratory Automation Types